
An American laboratory Just announced new results in “inertial” nuclear fusion, with an energy production of 1.3 megajoules. What does this advance represent for fusion, this “eternal” energy of the future?
Between the megaproject ITER, whose construction is progressing but which has had a difficult start, the projects launched by various countries, the private initiatives that are multiplying and which announce fusion reactors within 10 or 15 years, and the results obtained by the Lawrence Livermore National Laboratory on August 8, 2021, it is difficult to see clearly. Here is a quick overview to put all of this into perspective.
Magnetic or inertial confinement: two possible routes for nuclear fusion
There are two ways to use nuclear energy: fission, which is at work in current nuclear power plants, and fusion.
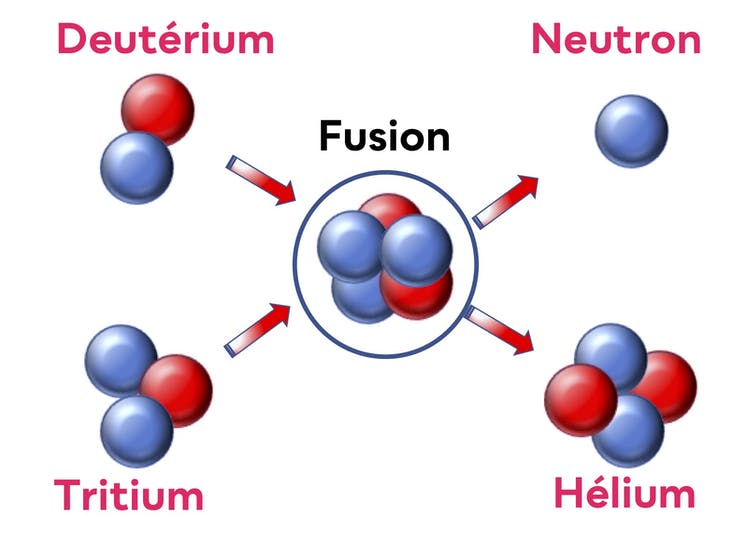
A fusion reactor is a power amplifier: the fusion reaction must produce more energy than is needed to heat the plasma to the required temperature and confine it. The current record was achieved in 1997 by the “Joint European Torus” or JET in the United Kingdom, where a power of 16 megawatts was generated by magnetic fusion, but it took 23 megawatts to trigger it.
Finally, obtaining a gain greater than 1 and demonstrating the feasibility of producing energy by fusion is a major objective of various ongoing projects.
There are two possible ways to achieve nuclear fusion: magnetic confinement, which uses powerful magnets to confine plasma for very long periods of time, and inertial confinement, which uses very powerful but very short lasers to compress the fuel and make it react. Historically, magnetic fusion has been preferred, because the technology required for inertial fusion (lasers in particular) was not available. The latter also requires much higher gains to compensate for the energy consumed by the lasers.
Inertial confinement
The two biggest projects are National Ignition Facility of the Lawrence Livermore National Laboratory (NIF) in the USA and the MegaJoule laser in France, whose applications are mainly military (nuclear explosion simulations) and financed by defense programs. The NIF is also pursuing research for energy.
The NIF uses 192 laser beams, with a total energy of 1.9 megajoules and a duration of a few nanoseconds, to trigger the fusion reaction using a so-called “indirect” approach. In fact, the fuel is placed inside a metal capsule of a few millimeters in size, which, heated by the lasers, emits X-rays. These heat and compress the fuel. The alignment of the lasers is easier than if they aimed directly at the target, but only part of their energy is converted into X-rays and used for heating.
The NIF has recently received strong media attention after a record energy production achieved on 8 August 2021. During this experience, an energy of 1.3 megajoules were produced, the highest value ever recorded by this approach.
The overall gain of 0.7 equals the record obtained by JET in 1997 by magnetic confinement, but if we look at the energy balance of the fuel itself (hydrogen target), we understand the excitement in this field. It has in fact absorbed 0.25 megajoules (laser-X-ray conversion causes losses) and generated 1.3 megajoules: fusion has therefore generated a good part of the heat necessary for the reaction, approaching ignition. A reactor will have to achieve much higher gains (greater than 100) to be economically interesting.
Magnetic confinement
Magnetic confinement is the preferred path for energy, as it offers better development prospects and benefits from greater feedback.
The vast majority of research focuses on the Tokamak, a configuration invented in the USSR in the 1960s where plasma is confined in the form of a torus by a powerful magnetic field. This is the configuration chosen by ITER, a demonstration reactor under construction in Cadarache in the south of France, whose objective is to demonstrate a gain of 10 — the plasma will be heated by 50 megawatts of power and must generate 500 megawatts of fusion power. While this titanic project involving 35 nations had a difficult start, construction is progressing at a steady pace and the first plasma is officially expected at the end of 2025, with a demonstration of fusion scheduled for the end of the 2030s.
The United Kingdom recently launched the STEP project (Spherical Tokamak for Electricity Production), which aims to develop a reactor connected to the grid in the 2040s. China continues with CFETR an ambitious program to demonstrate electrical and tritium production in the 2040s. Finally, after ITER, Europe plans a Tokamak demonstrator (DEMO) for the years 2050s, which implies deployment only in the second half of the century.
Also to read: ITER: how digital technology helps to avoid and control the instabilities of nuclear fusion
Another configuration — the stellarator — is being explored, in particular in Germany, with Wendelstein-7X which is showing very good results. If confinement in a stellarator is below what a tokamak can achieve, its intrinsic stability and recent results make it a serious alternative.
Private initiatives
In parallel with these public projects, we are hearing more and more about private initiatives, sometimes supported by big names like Jeff Bezos or Bill Gates. The oldest company (TAE) was founded in 1998 but an acceleration occurred after 2010 and in 2021 there were approximately one Thirty initiatives that attracted approximately $2 billion in total capital. The majority of these initiatives promise a reactor in the next 10 or 20 years and are presented as an alternative to the slowness of the traditional sector.

They use recent technological developments (high-temperature superconducting magnets for example), or various configurations, some of which had never really been explored: General Fusion uses, for example, pistons for compressing fuel. While the results are not always published in the scientific literature, we regularly see announcements showing real progress. If one of these companies were to demonstrate energy production within the promised timeframe, it could greatly accelerate the possibilities of using nuclear fusion.
A deployment that will take time
However, it should be borne in mind that the development of a first reactor is certainly extremely important, but that the deployment of a reactor fleet will take time. If we look at the deployment rates of photovoltaic, wind, and nuclear power, we see that in their exponential growth phase, the growth rate of installed capacity was between 20 and 35% per year. If we assume that the fusion manages to follow the same pace, we see that the fusion, following the ITER-DEMO line, could represent 1% of global energy demand (2019 value) around 2090. If we consider a reactor in the 2030s, this threshold could be reached around 2060 and fusion could play a bigger role in the second half of the century. Fusion therefore remains a long-term adventure.
Article published on The Conversation: linkage